Meet Zoey!
She traveled to Boston Children’s from Mississippi for care for a rare congenital condition called encephalocele. Learn more about her journey.
Meet Yah’ir!
Yah’ir is thriving after treatment for a complex heart defect at Boston Children’s. Read his incredible story.
Meet Sami!
Sami started life with both heart and throat surgeries — now he’s running and playing with his friends. Check out his story.
Ranked among the best pediatric hospitals in the nation
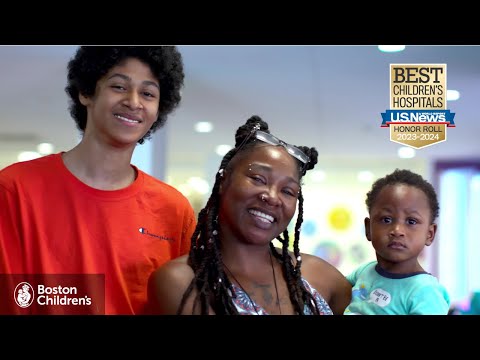
Watch as our CEO, Kevin B. Churchwell, MD, shares our gratitude for our ranking and our patients and families explain why they continue to choose Boston Children’s.
Best in Care
Did a staff member or volunteer make your family’s experience here at Boston Children’s Hospital better? Nominate them for a Best in Care award!
Stay connected!
Sign up for our weekly email newsletter for the latest parenting tips, patient stories, and news for your family from Boston Children’s.
Visit our Answers content hub
Visit our Answers content hub for all the latest stories from Boston Children's!
Para nuestras familias que hablan español
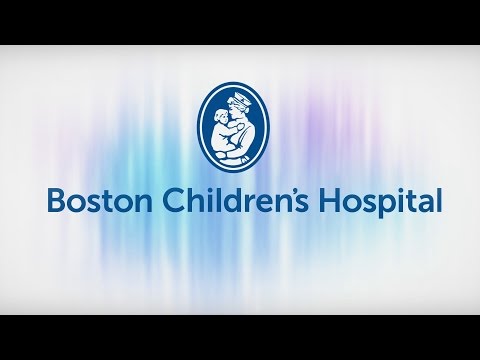
Desde la preparación para ser hospitalizado o para una cita ambulatoria, hasta la transición de vuelta a casa, el personal de Boston Children's Hospital está comprometido a crear una experiencia positiva para toda la familia.
Giving at Boston Children’s Hospital
Help children year-round
As a monthly partner, you are always there for patients and families. Your gift supports kids with chronic illnesses who need ongoing care.
Fundraise your way
Turn cupcakes into cures. Trivia into treatments. Dance parties into discoveries. Turn whatever you love into a fundraiser to help kids, because lifesaving answers can’t wait.
Walk to save lives
The path to healthy futures starts with you. Register for the Eversource Walk for Boston Children’s Hospital.